Upper Atmosphere
We study the atmospheric chemistry and dynamics or the mesosphere and lower thermosphere (MLT) regions. This work is largely in collaboration with Prof. John Plane (School of Chemistry) and is based on the Whole Atmosphere Community Climate Model (WACCM). WACCM extends from the surface to 140 km and has a detailed, state-of-the-art treatment of relevant physics and chemistry.
Impacts of Rockets and Space Industry
Iodine has the potential to cause stratospheric ozone depletion. Small satellites (<10 kg) in low Earth orbit require electric propulsion to prolong their time in orbit, and there is strong interest in replacing the rare gas propellant (Xe or Kr) with I2. In our paper Feng et al. (2023) we estimated the potential impact of thermosphere iodine injection from such satellites on stratospheric ozone. Since the demand for small satellites could increase greatly in the future, it is important to understand the potential risks to ozone depletion if iodine propulsion systems are used. Assuming a scenario in which 40,000 small satellites are launched each year into relatively low orbits and each satellite is assumed to contain 200 g I2, this could inject 8 tons/year of iodine ions into the upper atmosphere (above 120 km). Using the WACCM 3-D atmospheric chemistry-climate model, we showed that the perturbation to the total column ozone is very small, decreasing by 0.05 DU (<0.02%) globally and 0.2 DU (<0.1%) for September/October in the Antarctic polar region. However, a 100-fold increase in the number of launches and therefore mass of iodine emitted into near-Earth orbit is predicted to cause significant ozone depletion up to 14 DU (5.6%) in the Antarctic spring.
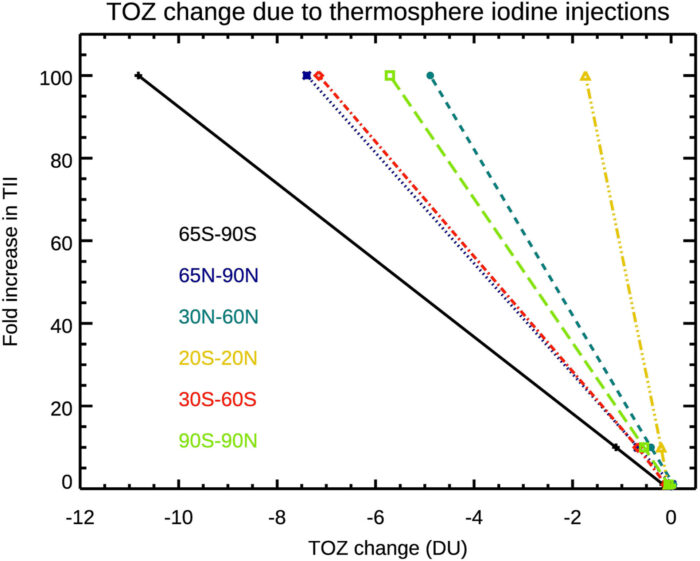
Annual mean total column ozone change (DU) with respect to the WACCM control simulation (CNTL) for different thermosphere iodine injection (TII) scenarios from small satellites over different latitude regions (see legend). Figure 4 from Feng et al. (2023).
Production of Nitrous Oxide
Nitrous oxide (N2O) is an important gas in the Earth's atmosphere - it is a greenhouse gas and leads to the production of other nitrogen species that can deplete the ozone layer. It was previously assumed to only be produced at the Earth's surface, primarily through bacterial processes in soil. However, satellite observations such as Atmospheric Chemistry Experiment-Fourier Transform Spectrometer (ACE-FTS) have revealed a minor upper atmospheric source in the MLT.
In Kelly et al. (2018) we reported the development of a mechanism and the first model simulations, which can explain the formation of this MLT N2O layer. N2O production occurs primarily via a reaction route involving the excitation of N2 from secondary electrons. Simulations using the WACCM, with external forcing from the Global Airglow model, quantitatively reproduce the observed vertical, latitudinal, and seasonal N2O variations. Sensitivity results indicate that photoelectrons are far more important than previously predicted, causing approximately two thirds of global N2O production in the MLT. Energetic electron precipitation over high latitudes provides the remaining contribution.
Our results indicate that N2O is constantly made through a chemical process involving the arrival of electrons trapped by the Earth's magnetic field near the poles, and light from the Sun at all latitudes. However, it should be emphasized that the concentration of N2O in the upper atmosphere is extremely small compared to the concentration of N2O in the lower atmosphere.
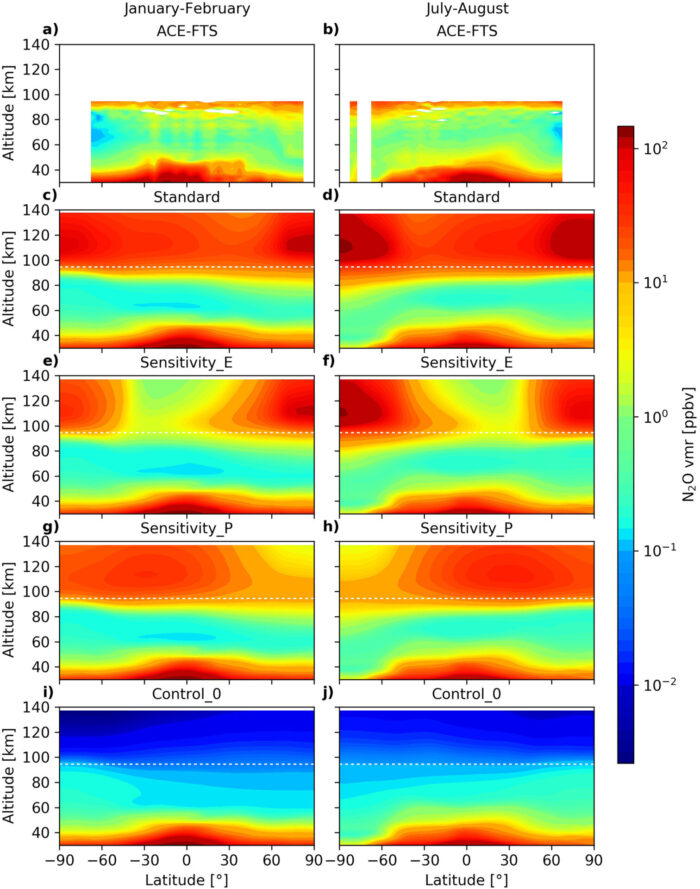
Top panel: Latitude-height zonal mean cross sections of N2O vmr (ppbv) averaged for (left column) January–February and (right column) July–August. Panels (a) and (b) show ACE-FTS satellite data for 2013, which extends up to 94.5 km. Panels (c)–(j) show four corresponding WACCM simulations, where each y axis uses the simulated geopotential height for direct model comparisons. The horizontal dashed white line indicates 94.5 km. Figure 1 from Kelly et al. (2018).
Mesospheric Metal Layers
We have also developed WACCM to include metal layers produced by ablating meteors (e.g. Feng et al., 2013) and compared them with satellite observations (e.g. Dawkins et al., 2014, 2015).
References
Dawkins, E.C.M., W. Feng, M.P. Chipperfield and J.M.C. Plane, The near-global mesospheric potassium layer: Observations and modelling, J. Geophys. Res., 120, 7975-7987, doi:10.1002/2015JD023212, 2015.
Dawkins, E.C.M., J.M.C. Plane, M.P. Chipperfield, W. Feng, J. Gumbel, J. Hedin, J. Hoffner and J.S. Friedman, First global observations of the mesospheric potassium layer, Geophys. Res. Lett., 41, 5653-5661, doi:10.1002/2014GL060801, 2014.
Feng, W., J.M.C. Plane, M.P. Chipperfield, A. Saiz-Lopez and J.-P. Booth, Potential stratospheric ozone depletion due to iodine injection from small satellites, Geophys. Res. Lett., 50, e2022GL102300, doi:10.1029/2022GL102300, 2023.
Feng, W., D.R. Marsh, M.P. Chipperfield, D. Janches, J. Hoffner, F. Yi and J.M.C. Plane, A global atmospheric model of meteoric iron, J. Geophys. Res., 118, 9456-9474, doi:10.1002/jgrd.50708, 2013.
Kelly, C.W., M.P. Chipperfield, J.M.C. Plane, W. Feng, P.E. Sheese, K.A. Walker and C.D. Boone, An explanation for the nitrous oxide layer observed in the mesopause region, Geophys. Res. Lett., 45, 7818-7827, doi:10.1029/2018GL078895, 2018.